Thermal Energy Storage (TES) is an energy storage method that can help balance energy demand and supply daily, weekly, and even seasonally. TES refers to heating or cooling a medium to use the energy when required later. The most common application of TES systems is integration with solar systems. Further, other application areas include storing heat in building structures, waste heat and district heating systems, and two heat pumps and combined heat and power (CHP) generators in district heating networks.
Importance of Thermal Energy Storage
Consumer awareness, environmental agencies, and government policies focus on renewable energy sources with reduced GHG emissions. Solar panels, wind, and tide energy systems are among the best possible conventional energy source (fossil fuel) alternatives. However, these systems do not always generate energy simultaneously. Thermal energy storage seems to be the answer.
This particular aspect can be complemented by energy storage methods, e.g., by integrating TES with conventional renewable sources. Thermal energy storage (TES) is much cheaper than electricity storage, and it has a high potential of integrating intermittent resources via, e.g., heat pumps or electric boilers.
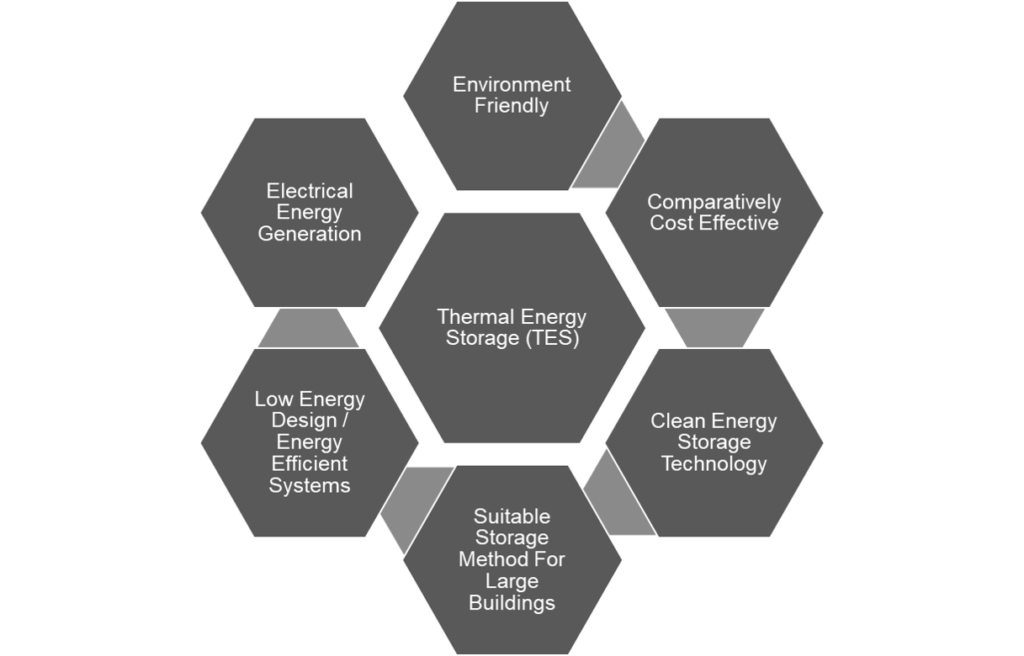
Market Insight
The Global Thermal Energy Storage market size was estimated at US$ 5.6 Billion in 2021. Further, it is expected to reach $10.5 billion by 2027, rising at a market growth of 10.4% CAGR from (2022-2027).
Renewable energy, primarily concentrated solar power, and the growing demand for HVACs (for large-scale heating and cooling). They are key factors driving the industry’s growth. Concentrated solar power (CSP), when integrated with thermal energy storage systems, offers grid flexibility. Also, it minimizes efficiency losses by generating electricity through dry cooling during lower ambient temperatures.
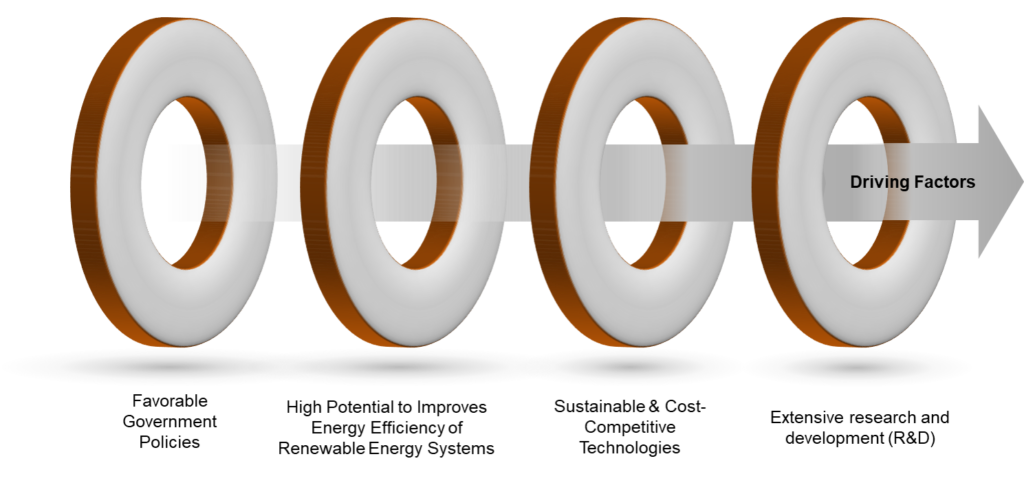
Fig 2: Driving Factors of Thermal Energy Storage
As the technology is still developing, the high cost of research and development to achieve better energy efficiency and the high installation cost are growth barriers in the domain. The TES market can be divided into three storage types: sensible, latent, and thermochemical heat.
Types of Thermal Power Storage
Sensible heat storage (SHS) is the most viable thermal energy storage option. It is based on storing thermal energy by heating or cooling a liquid or solid storage medium (e.g., water, sand, molten salts, or rocks) and has several residential and industrial applications.
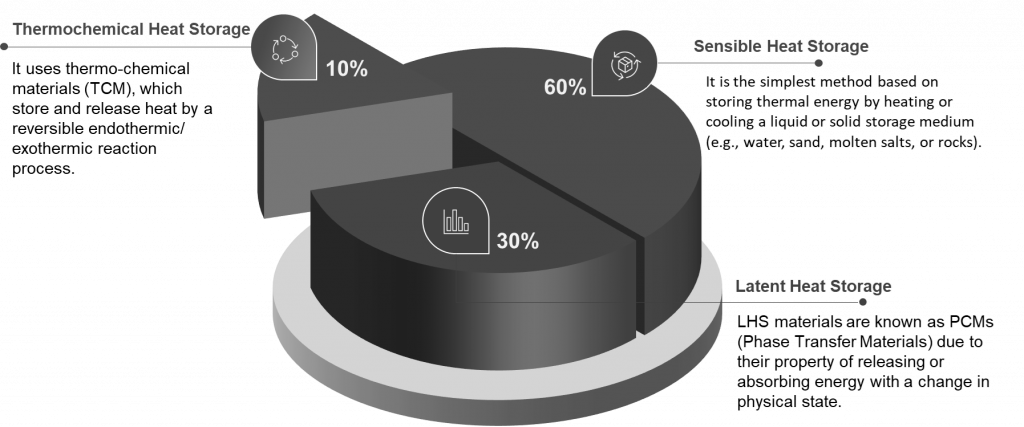
Latent heat storage (LHS) or phase change materials (PCMs) mainly store heat in the phase-change process (at a quite constant temperature). They are directly connected to the substance’s latent heat. These materials can release or absorb energy with a change in physical state. PCMs are an effective way of storing thermal energy and have the advantages of high-energy storage density and the isothermal nature of the storage process.
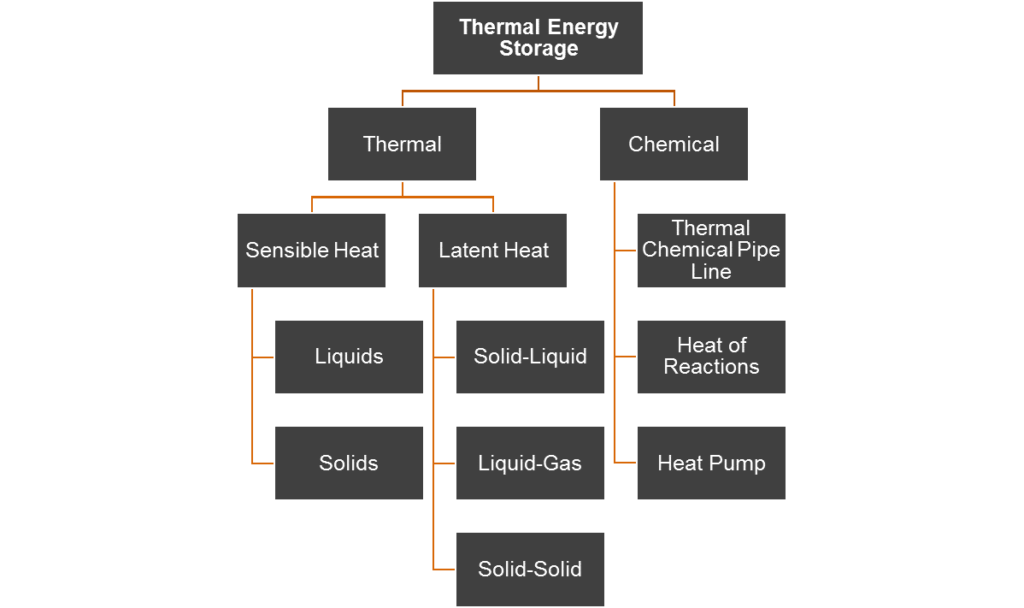
PCMs can be divided into low-temperature (Ice, Water gel), medium-temperature (hydrated salts, polymer materials), and high-temperature (molten salts, metal alloys) PCMs. Thermochemical energy storage (TCES) works through a cycle’s endothermic and exothermic chemical reactions. Compared to sensible heat and latent heat storage, this theoretically offers higher energy density with minimum energy loss during long-term storage due to temperature-independent storage.
Nano-engineered Phase Change Materials (NEPCMs)
NEPCMs are a homogeneous mixture of nanoparticles dispersed in PCMs. Depending on the structure and nature of the nanoparticles used, they improve the PCM’s thermo-physical properties. They can be used in thermal energy storage systems that require rapid thermal cycle times, such as building heating and cooling, electronics cooling systems, refrigerators, etc.
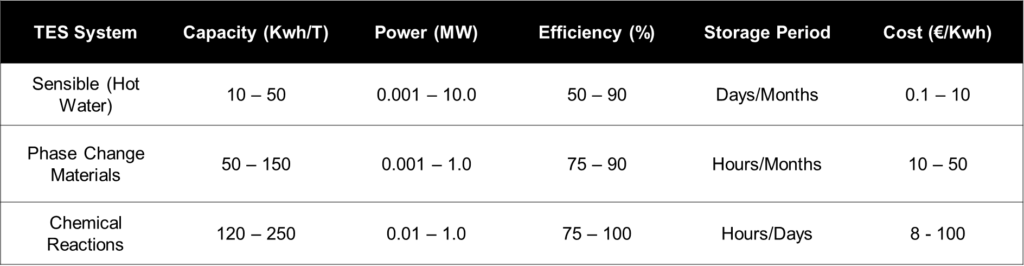
Carbon nanotubes, nanofibers, graphite, graphene, metallic, and metal oxide nanoparticles are some examples of nanoparticles commonly dispersed in PCMs. A solid-liquid phase transition process can be nano-engineered to improve the latent heat of phase change or increase the heat transfer rate in either state. Adding nanoparticles to PCMs increases the energy level of PCMs, reduces the fluctuations in melting temperature, and increases the latent heat of samples throughout multiple thermal cycles.
The overall thermos-physical properties and fundamental physical characteristics of the configuration significantly influence the performance of the PCMs. For example, the location and number of nucleation sites.
Solid-liquid phase change materials (PCMs) have been integrated with a range of thermal systems have been systems to improve operations, e.g.-
- PCM-integrated heat pump provides continuous heating without drawing on the electrical grid during peak load periods.
- PCM-integrated ground heat exchangers of ground-source heat pumps result in higher coefficient of performance (COP) values.
- PCM-integrated building cooling applications have been quite effective in passive mode or in conjunction with air conditioning systems.
Novel Technologies for Heat Storage
Miscibility Gaps Alloy (MGA) block developed by an Australian clean energy company, MGA Thermal, got AUS$1.27 million in funding from the Australian Renewable Energy Agency (ARENA) to scale up its thermal energy storage technology.
Novel low-cost thermal storage (Molten Salt Storage): Abengoa, Spain, is planning to use low-cost Photovoltaic power to support new molten salt thermal energy storage systems at parabolic trough CSP plants. This installation’s cost efficiency is very low, about $71/MWh, as demonstrated by Morocco’s Noor Midelt I hybrid CSP-PV project.
Molecular solar thermal energy storage (MOST): Researchers from Chalmers University of Technology, Sweden, and Shanghai Jiao Tong University, China, developed an ultra-thin chip as a thermoelectric generator. This chip can provide a radical way of generating electricity from solar energy. The captured solar energy can be stored for about 18 years before converting to electricity. Here, the solar energy is stored as chemical energy and released as heat. Further, the micro-fabricated thermoelectric generators produce electricity from this heat.
Thermal Energy Storage: Business Ecosystem
US-manufactured thermal energy storage tanks (TES tanks) produce ice or chilled water during off-peak hours. They store it in an insulated tank and use it to cool any facility during peak hours. These tanks offer lower energy costs, capital outlays, and maintenance costs.
Abengoa Solar, Spain, is installing its Solar Thermal Electric (STE) Power Plant for direct electric generation in Latin America. The Chilean Ministry of Energy and the Production Promotion Corporation (CORFO) awarded this project to Abengoa via an international tender. Moreover, the solar plant, with a 110 MW capacity, will store thermal energy in molten salts for 18 hours.
Abengoa’s project in Chile will prevent the emission of approximately 643.000 T CO2, equivalent to the emissions produced by 357,000 vehicles driving ceaselessly for one year.
Evapco, US, has a proprietary thermal ice storage unit (Extra-Pak® Ice Coil). These ice coils are constructed of high-quality steel and hot-dip galvanized after assembly. They are suitable for all types of large, energy-saving thermal storage systems with field-constructed concrete tanks.
Other important players include Baltimore Aircoil Company, US; Brightsource Energy, US; Steffes, US; Terrafore Technologies, US; Ice Energy; and Cryogel.
Conclusion
Thermal Energy Storage technology is developing and needs more research and development to achieve high efficiency. Higher efficiency is currently a challenge due to high installation costs.
NEPCMs offer improved efficiency of thermal energy storage systems. However, a few limitations (e.g., poor thermal conductivity) must be addressed to obtain optimal performance. Further, some NEPCMs undergo rapid structure and thermal performance changes when subjected to repeated charging/discharging cycles (thermal cycling). The adoption of thermal energy storage technologies can ensure an uninterrupted energy supply. Thermal energy storage technologies also support the integration of various renewable energy sources, including wind and solar, into the electricity grid.