Microbial Fuel Cells: Electricity from Wastewater
Currently, most industries or domestic houses use electricity produced from non-renewable sources like coal, petrochemical products, etc. The continuous depletion of natural or non-renewable energy resources has led the world to a stage of global energy crisis. To avoid such a crisis in the future, researchers are developing technologies for generating energy from alternative and sustainable sources. In the race to develop alternative fuel or energy from sustainable and renewable energy resources, Microbial Fuel cells (MFCs) have emerged as pioneering technology that offers the dual benefit of wastewater treatment and power generation.
Understanding Microbial Fuel Cell
Microbial Fuel Cells use the ability of electrogenic microbes to oxidize organic contaminants present in the wastewater and produce bioelectricity. The chemical energy present in chemical bonds of organic matter of various substrates such as industrial wastewater, municipal waste, lignocellulosic biomass, brewery discharge, starch processing wastewater, acetate, glucose, etc., using the catabolic abilities of microorganisms. In the Microbial Fuel Cells, there are two major components: separated by a proton exchange membrane, one is an anode for oxidizing the organic matter, and the second is a cathode for reduction to generate pure water. The electricity is generated due to the continuous biological oxidation of organic substrate in the anodic chamber and oxygen reduction in the cathodic chamber. Microorganisms used for generating electricity act as biocatalysts to decompose organic substrates and generate electrons and protons through anodic oxidation. The transfer of generated electrons via an external circuit and protons through the proton exchange membrane (PEM) results in a reduction reaction with oxygen to generate water in the cathode region.
An example denotes electrode reactions in the conventional MFC using acetate as a substrate.
- Anodic reaction: CH3COO– + H2O→ CO2 + 2 H+ + 8e–
- Cathodic reaction: O2 + 4e– + 4 H+ →2 H2O
The use of MFCs in wastewater treatment and energy generation offers many advantages, such as energy efficiency, mild production conditions, simple operation, sustainable technology, a reduction in the energy footprint of wastewater treatment processes, and the availability of a variety of biocatalysts for catalyzing organic matter.
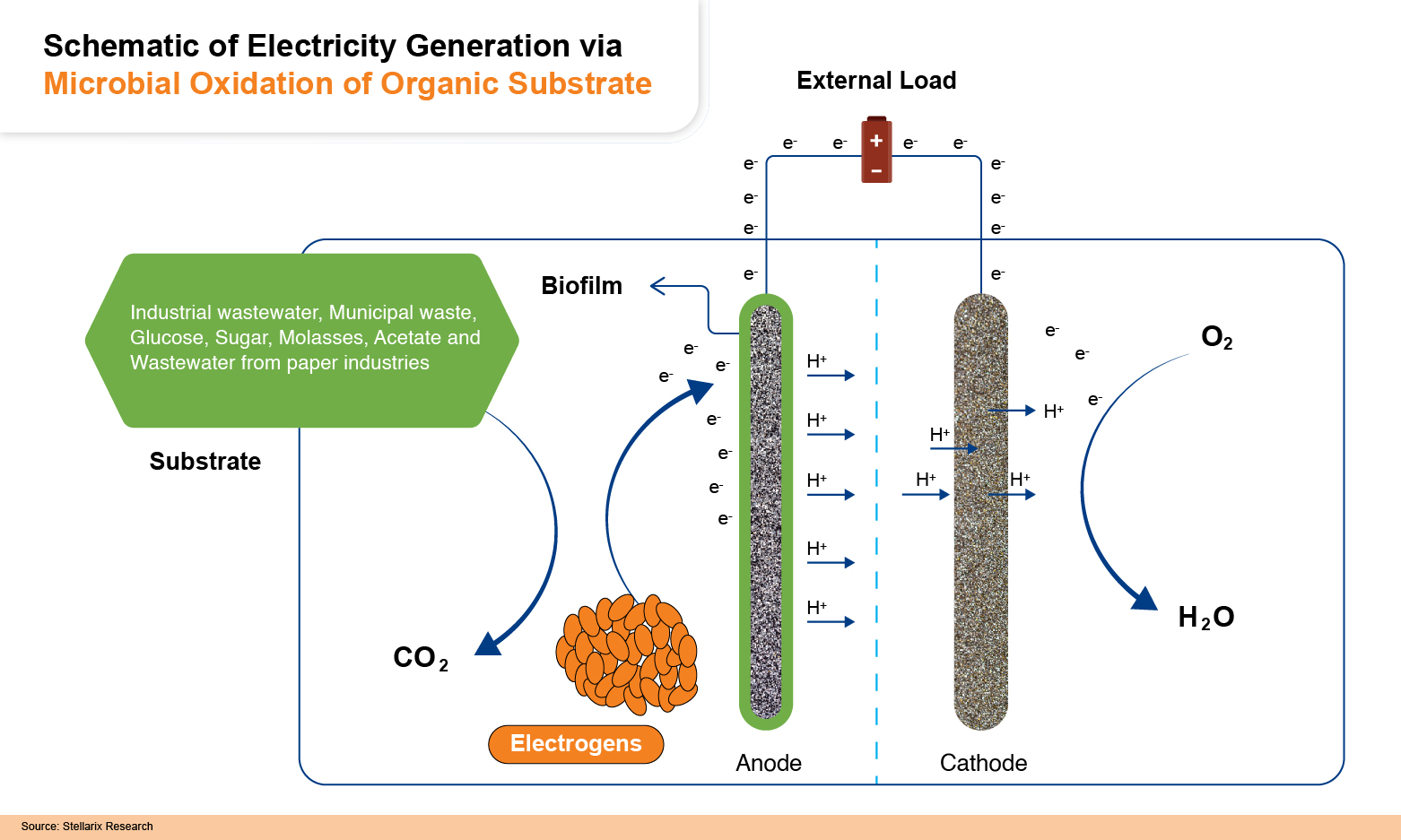
Challenges to MFCs Commercialization
It has always been suggested that Microbial Fuel Cells are ecologically sustainable alternative techniques for generating electricity while removing pollutants from various forms of wastewater; however, many challenges stand between Microbial Fuel Cells and their commercialization for wastewater treatment. It has been suggested that Microbial Fuel Cells are ecologically sustainable alternative techniques for generating electricity while removing pollutants from various forms of wastewater; however, many challenges stand between Microbial Fuel Cells and their commercialization for wastewater treatment. Challenges include limited electricity generation, high costs required for operating and maintaining MFCs, scalability issues, competitive microbial reactions, optimized designs for higher efficiencies, etc. For instance, the increased cost of the MFC system is due to the use of expensive electrode materials, such as catalysts, current collectors, and separator materials.
What Advancements Are Stepping In?
To explore the commercial potential of MFC technology, one has to consider measures such as minimization of high-cost operation, improving poor power generation, regulating microbial performance in the unit, and creating a full-scale MFC model. Researchers are working on several modifications in terms of the use of alternative microorganisms (biocatalysts), bacterial gene alterations, alternative membranes, anodic surface modifications, and other modifications to boost the power output and optimize the efficiency of MFCs for wastewater treatment.
1. Anode Materials
MFC performance and its power density output depend on how and what type of anode we select. Anode has a very significant role to perform in forming a stable biofilm for microbial adhesion and enhancing conductivity through efficient electron transfer by offering increased surface roughness/ areas for higher power density. To enhance the efficiency of anode materials, nanostructure materials have become the first choice of researchers for fabricating advanced electrodes since they offer high specific surface areas, increased transfer rates, biocompatible, and low cost with ease of fabrication. Advanced anode materials include metal nanoparticles (Cu, Au, Pt, Pd, & Ag), quantum dots, metal oxide, carbonaceous materials, and nanocomposite materials. Carbon-based anode materials such as graphene, carbon nanotubes, carbon paper/ cloth, etc., are most commonly used because of enhanced electrical conductivity, biocompatibility, and relatively inexpensive. The most efficient anode materials developed to date with the highest power density output are based on the graphene and stainless steel composite structure, such as Graphene-modified stainless steel mesh with a power density output of 2668 mW.m-2 and its nanocomposite with a power density output of 2000 mW.m-2.
2. Cathode Materials
In optimizing the cost and performance of microbial fuel cells, selecting low-cost cathode materials with high capacity of cathode-based oxygen reduction is important. Common cathode materials include air cathodes, aqueous air cathodes, and bio-cathodes. Generally, cathodes are made of carbon-based electrodes and supported by a layer of a catalyst such as platinum, titanium, ferricyanides, etc., which makes cathodes highly expensive and unstable for large-scale use. The problem can be addressed using cost-effective carbon-based alternatives such as stainless steel, carbon paper, carbon cloth, etc., or using biocathodes, which are very inexpensive alternatives.
Currently, research on nanocomposite catalysts to enhance electrochemical performance has been evolving to highly expensive platinum and titanium catalysts. Advancements in nanocomposite include catalysts based on metals, metal oxides, and combinations with non-metal materials. Examples include Cu2O-modified activated carbon, α-MnO2 nanowires, MnO2-reduced graphene oxide (GO) composites, and Iron(Ⅱ) phthalocyanine (FePc) – GO.
3. Membrane Materials
Membranes in MFCs transfer protons generated via anodic oxidation to a cathodic chamber and prevent oxygen diffusion from cathodic chambers to anodic chambers. Its presence maintains long-term efficiency in the MFCs and prevents a reduction in Columbic efficiency. The main challenges in conventional membranes include expensive materials, high cost, sensitivity to fouling, and increased internal resistance. Researchers are employing ion exchange and porous membranes to address the listed problems. Nafion, from DuPont, is a common Cation Exchange Membrane (CEM) due to its high proton conductivity.
- Cation Exchange Membrane (CEM): CEM transports positive-charge ions, such as protons, and fixed negative changes, for example, Nafion, Zifrons, Hyflon, etc.
- Anion Exchange Membrane (AEM): AEM transports negatively charged ions while fixing positively charged ions. It generates a higher current than CEM because hydroxyl ions consume the generated protons in the anode chamber. In addition, AEM prevents an acidic environment in the anode chamber. As a result, ion transport is increased at the anode, and resistance is decreased at the cathode.
- Bipolar Membrane (BPM): BPM has unique characteristics, as it contains both AEM and CEM, which allow the transport of both H+ and OH- ions over the membrane. However, it faces issues with pH gradients.
- Porous Membrane: Proton exchange membranes based on porous membranes are extremely cost-effective alternatives to conventional ones. Ions are separated based on the pore size. Common examples employed in MFCs include Glass Wools, Ultrafiltration Membranes (UFMs), and Microfiltration Membranes (MFMs).
Ecosystem Activities and Future Perspective
Researchers have been working on developing electricity generation through microbial cells for decades. Despite the successful implementation on a laboratory scale, MFC technology is struggling to move forward because of its inherent low power output. It is said that “A total of 300 bn m3/year of domestic wastewater generated worldwide contains an estimated 600 bn kWh of energy”. If the scientific community succeeded in commercializing MFC at a larger scale, it could produce energy enough to power the European Chemical Industry for a Year”. The most significant limiting factors in commercializing the MFC are optimizing the bacterial activity at the anode and the limitation of continuous oxygen supply at the cathode for larger-scale units. Players have been trying to overcome challenges, including Aquacycl, MICROrganic Technologies, the University of Bath, and collaborative activities between Pennsylvania State University and Tobyhanna Army Depot in Pennsylvania.
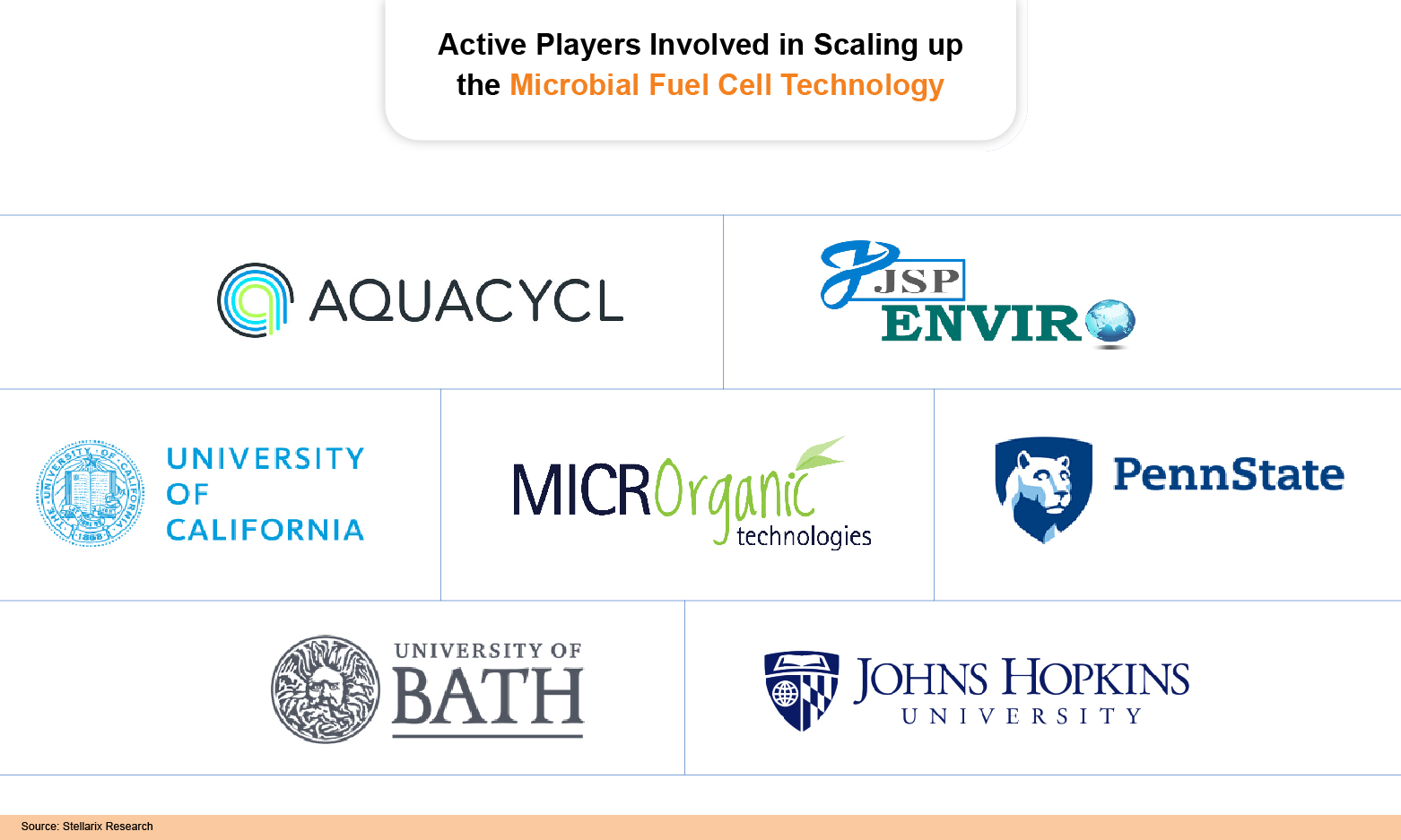
- Aquacycl
Aquacycl is a US-based start-up that stepped into the market with unique Microbial Fuel Cell Technology, utilizing a modular approach for Wastewater Treatment with low or net zero energy requirements. The technology offered by Aquacycl, BETT (BioElectrochemical Treatment Technology), helps in treating wastewater while recovering energy as direct electricity. The company utilizes a series of MFCs, each component being the size of a car battery. Aquacycl CEO Orianna Bretschger explains that the system is better suited for small volumes with highly concentrated waste streams (> 25,000 BOD) and less suited for large volumes because of their small size. One of Aquacycl’s commercial operations is running at PepsiCo’s bottling facility in Fresno, California, US, where they were able to mitigate more than 800 tons of CO2 in just one year.
- MICROrganic Technologies
MICROrganic Technologies offers VIVA MFC Technology for treating wastewater from high-strength industrial to low-strength municipal waste. The technology has excellent characteristics, including real-time monitoring (it can diagnose biological upsets and mechanical issues down to an individual electrode), a reduction in energy use of 85-90%, the generation of clean DC power in addition to energy storage, and efficient nutrient recovery.
- JSP Enviro
JSP Enviro, an India-based start-up founded by an IIT Madras alumni, plans to establish a prototype microbial fuel cell technology by collaborating with a garment dyeing unit in Tirupur.
- Pennsylvania State University
Researchers at Pennsylvania State University piloted a 1400 L microbial fuel cell with air cathodes at the Tobyhanna Army Depot in Pennsylvania. It could make up to remove 70% of biochemical oxygen demand and turn just 9% of microbial electrons into electricity.
- Others Active Players
Players are working on developing soil-based MFC since soil can act as a source of exoelectrogenic microbes and as the source of fuel (the electrolyte and the separator between anode and cathode), eliminating the need for membranes/ pumps. The University of Bath and the University of California aimed to produce electricity using soil-based MFC to apply water disinfection and sensors for agriculture, respectively.
Conclusion and Future Perspective
Microbial Fuel Cells seem to be a groundbreaking technology that addresses energy crisis, sustainability, and wastewater management issues. Since conventional wastewater treatment technologies are energy-intensive, using MFC technology as a sustainable wastewater treatment alternative offers researchers exceptional opportunities. Despite the enormous potential in sustainable energy, scaling MFC technology to a large-scale, low-cost application with excellent power output is still challenging. The technology still needs a detailed investigation into aspects such as developing innovative materials, structural configurations, and more efficient microbial species, considering cost and efficiency. The successful introduction of Aquacycl’s BioElectrochemical Treatment Technology (BETT) opens up many more opportunities for leaders in wastewater treatment and other relevant industries to develop novel solutions for exploring MFC scalability.